Contents 1 Overview
2
2 The Leica AT401 Absolute Tracker
2
3 The CERN Coordinate System 3.1 Conversion from CERN to Miniball coordinate system . . . . . . . . . . . . . . . . . . . . . . 3.2 Conversion from Miniball to CERN coordinate system . . . . . . . . . . . . . . . . . . . . . .
2 4 4
4 Alignment of the frame
4
5 Alignment of the Coulex target chamber
6
6 Alignment of the targets
7
7 Alignment of the SPEDE target chamber
8
1
1
Overview
In the past, we used markings on the floor and on the wall behind the bending magnet before Miniball (viewed by opening a port-hole in the magnet) to set up the theodolite, so that it was directly over the point on the floor and looking at the point on the wall. This meant the theodolite was looking along the direction exactly opposite the beam direction. So if the target was centred on the crosshairs of the theodolite, it was correct. With HIE-ISOLDE it is no longer possible to open up a port-hole in the magnet and there is, in any case, no line of site to the wall and no mark on the wall. Instead there is a mark at the point where the beam intersects the wall after Miniball. There is also a mark on the floor after the beam dump. So in principle, we could mount the theodolite over the mark on the floor, line up to the one on the wall and rotate 180◦ and we would be looking also as before. Note that there are two ways to rotate the theodolite through 180◦ : • rotating about the vertical axis and using the scale to determine the number of degrees to rotate • rotating just the telescope about the horizontal axis and using the spirit level to get it level before and after the rotation. However, in 2015, we tried a new method for aligning, with the help of the alignment people (Michal Rebisz, Antje Behrens and Benoit Cummer). This new method is described in this document.
2
The Leica AT401 Absolute Tracker
The alignment service have a Leica AT401 Absolute Tracker, which is first set up using known points fixed in the floor and walls at ISOLDE, where they mount prisms and the AT401 uses a laser to determine is position relative to these points. After that, they can place a prism at any point and determine its absolute position in the CERN Coordinate System.
3
The CERN Coordinate System
The CERN Coordinate System has its origin at the PS, but there are offsets of about 2 km added to each coordinate, so it cannot be confused with any other system. The coordinates are either given as xCCS , yCCS , and zCCS or xCCS , yCCS , and HCCS . The relationship between zCCS and HCCS is given by:
zCCS = HCCS + 2000.00079 −
d2 2(R + h)
where
d=
p
(xCCS − 2000)2 + (yCCS − 2097.7927)2
and R is the mean radius of the earth in metres given by
R = 6371000 To go in the other direction, we use 0010
hCCS
zCCS − 2000.00079 + 0001 = d cos R
d2 2R
0011
Using the MADX calculation from 15/06/2015, which is in version 1.1 of the EDMS document 1323585 (https://edms.cern.ch/document/1323585/1.1) in the file XT01 survey ccs.txt. we have the coordinates of the Miniball target position:
x=1864.28940, y=2282.82486, z=2437.48881, h=437.49215 [m] 2
Figure 1: The Leica AT401 Absolute Tracker.
3
We define a Miniball coordinate system with the origin at the nominal target position, the y direction in the direction of the beam and the z-direction vertically upwards. So the z-axis is in the same direction as that of the CERN one, but it is rotated by -257.85485997◦ = -4.5004162987 radians1 , relative to the CERN coordinate system. Note, however, that we use millimetres not metres.
Angle between coordinate systems = -257.85485997◦ = -4.5004162987 radians Note that the Miniball CAD diagram is offset by 25 mm relative to the Miniball coordinate system. This is because it was designed for a nominal beam height of 1750 mm, but at ISOLDE the beam height is nominally at 1725 mm.
3.1
Conversion from CERN to Miniball coordinate system xM B =
1000 · (0.97761779 · (yCCS − 2282.824858) − 0.21038884 · (xCCS − 1864.289396))
yM B =
1000 · (−0.97761779 · (xCCS − 1864.289396) − 0.21038884 · (yCCS − 2282.824858))
zM B =
1000 · (zCCS − 2437.488812)
or 1000 · (HCCS − 437.492155)
zM B = where
0.97761779 = sin(−257.854860◦ ) 0.21038884 = cos(−257.854860◦ ) where the values with the CCS subscript are in the CERN Coordinate System and those with the MB subscript are in the Miniball coordinate system. The Miniball coordinate system has its origin at the nominal target position, with the y direction representing the beam and x horiziontal and z vertical.
3.2
4
Conversion from Miniball to CERN coordinate system xCCS =
1864.289396 − 0.97761779 · yM B /1000 − 0.21038884 · xM B /1000
yCCS =
2282.824858 + 0.97761779 · xM B /1000 − 0.21038884 · yM B /1000
zCCS =
zM B /1000 + 2437.488812
HCCS =
zM B /1000 + 437.492155
Alignment of the frame
To align the frame, we determined the absolute coordinates of the rings onto which the arcs are attached. There are three such rings below the target chamber and three above,on each side. By measuring at least three points on a ring, they can fit a circle and determine the centre of the circle. This should be exactly below or above the nominal target position. From the CAD diagrams, we know the positions of the centre of the top and bottom faces of each ring. If we number the rings from top to bottom, so the highest ring is ring 1 and the lowest is ring 12, we have the following coordinates for the centre of the top and bottom flat surfaces of each ring: 1 Angle
also taken from EDMS document 1323585
4
Figure 2: The Miniball frame, showing the 12 rings (bright green), numbered 1 to 12 from top to bottom. The beam goes into the page from left to right. Ring 1 1 2 2 3 3 4 4 5 5 6 6 7 7 8 8 9 9 10 10 11 11 12 12
Surface Top Bottom Top Bottom Top Bottom Top Bottom Top Bottom Top Bottom Top Bottom Top Bottom Top Bottom Top Bottom Top Bottom Top Bottom
xM B , yM B , zM B [mm] 0, 0, +835 0, 0, +795 0, 0, +795 0, 0, +755 0, 0, +755 0, 0, +717 0, 0, +585 0, 0, +547 0, 0, +547 0, 0, +507 0, 0, +507 0, 0, +467 0, 0, -473 0, 0, -513 0, 0, -513 0, 0, -552 0, 0, -552 0, 0, -591 0, 0, -723 0, 0, -761 0, 0, -761 0, 0, -801 0, 0, -801 0, 0, -841
xCCS , yCCS , zCCS , HCCS [m] 1864.28940, 2282.82486, 2438.32381, 438.32715 1864.28940, 2282.82486, 2438.28381, 438.28715 1864.28940, 2282.82486, 2438.28381, 438.28715 1864.28940, 2282.82486, 2438.24381, 438.24715 1864.28940, 2282.82486, 2438.24381, 438.24715 1864.28940, 2282.82486, 2438.20581, 438.20915 1864.28940, 2282.82486, 2438.07381, 438.07715 1864.28940, 2282.82486, 2438.03581, 438.03915 1864.28940, 2282.82486, 2438.03581, 438.03915 1864.28940, 2282.82486, 2437.99581, 437.99915 1864.28940, 2282.82486, 2437.99581, 437.99915 1864.28940, 2282.82486, 2437.95581, 437.95915 1864.28940, 2282.82486, 2437.01581, 437.01915 1864.28940, 2282.82486, 2436.97581, 436.97915 1864.28940, 2282.82486, 2436.97581, 436.97915 1864.28940, 2282.82486, 2436.93681, 436.94015 1864.28940, 2282.82486, 2436.93681, 436.94015 1864.28940, 2282.82486, 2436.89781, 436.90115 1864.28940, 2282.82486, 2436.76581, 436.76915 1864.28940, 2282.82486, 2436.72781, 436.73115 1864.28940, 2282.82486, 2436.72781, 436.73115 1864.28940, 2282.82486, 2436.68781, 436.69115 1864.28940, 2282.82486, 2436.68781, 436.69115 1864.28940, 2282.82486, 2436.64781, 436.65115
Note, however, that these values are only achieved if the ball is closed and the locking pins are in place. However, it is rather hard to measure in this configuration, so it may be necessary to compromise. The rings above the target chamber are most affected by the absence of the locking pins.
5
5
Alignment of the Coulex target chamber
Miniball Coulex target chamber Beam direction
10
11 3
2
12
9
Coordinates relative to
CERN Coordinates System in m:
to centre in mm: 0
= (0,
1
0,
0)
0
(1864.2894, 2282.8249,
437.4922)
= (-137.5, -367.5, 100)
1
(1864.6776, 2282.7678,
437.5921)
2
= (-137.5, +367.5, 100)
2
(1863.9590, 2282.6131,
437.5922)
3
= (+137.5, -367.5, 100)
3
(1864.6197, 2283.0366,
437.5922)
4
= (+137.5, +367.5, 100)
4
(1863.9012, 2282.8820,
437.5922)
5
= (-127.5, -367.5, 100)
5
(1864.6755, 2282.7775,
437.5921)
6
= (-127.5, -351.5, 100)
6
(1864.6599, 2282.7742,
437.5921)
7
= (+127.5, -351.5, 100)
7
(1864.6062, 2283.0235,
437.5922)
8
= (+127.5, -367.5, 100)
8
(1864.6218, 2283.0268,
437.5922)
9
= (-127.5, +351.5, 100)
9
(1863.9726, 2282.6263,
437.5922)
10 = (-127.5, +367.5, 100)
10 (1863.9569, 2282.6229,
437.5922)
11 = (+127.5, +367.5, 100)
11 (1863.9033, 2282.8722,
437.5922)
12 = (+127.5, +351.5, 100)
12 (1863.9189, 2282.8756,
437.5922)
0
6
7 4
1 5
8
Figure 3: The target chamber alignment There are two ways to determine the alignment of the chamber:
6
Figure 4: The Leica retro-reflective targets mounted on the Miniball target wheel • Using the upper corners of the front and back plates • Using the sphere of the chamber itself The former method relies on the information in figure 3. In practise, it is not possible to use the points on the side plates, as there is a small difference depending how the side plates are mounted. However, you must have the side plates on to change the alignment, otherwise you could damage the target chamber. So it is really points 6, 7, 9 and 12 which need to be used. Point (See fig. 3) 6 7 9 12
xM B , yM B , zM B [mm] -127.5, -351.5, 100 +127.5, -351.5, 100 -127.5, +351.5, 100 +127.5, +351.5, 100
xCCS , yCCS , zCCS , HCCS [m] 1864.65985, 2282.77416, 2437.48881, 437.59215 1864.60620, 2283.02346, 2437.48881, 437.59215 1863.97259, 2282.62626, 2437.48881, 437.59215 1863.91894, 2282.87555, 2437.48881, 437.59215
The latter method involves measuring several points on the outer surface of the sphere and fitting a sphere to those points. For this, the side plates need to be removed (at least on one side), so it is not feasible to adjust the chamber this way, but it is a good check after using the other method.
6
Alignment of the targets
There are two ways to do this: • set up the theodolite so that it is looking in the inverse direction of the beam and check the crosshairs of the theodolite are centred on the target • use the absolute method with some special Leica retro-reflective targets (see fig. 4) mounted on the target wheel. 7
The former method has the disadvantage that it doesn’t measure the offset of the target along the beam direction. The nominal target position is2 : Point (See fig. 3) 0
7
xM B , yM B , zM B [mm] 0, 0, 0
xCCS , yCCS , zCCS , HCCS [m] 1864.28940, 2282.82486, 2437.48881, 437.49215
Alignment of the SPEDE target chamber
Figure 5: The SPEDE chamber The SPEDE target chamber is 27 mm longer than the Coulex one. As with the Coulex chamber, the target is centred between the two flanges, which are compatible with the Coulex ones. The points in the following table correspond to points 0, 5-11 in 3. i.e. without the side plates. The width (±127.5 mm) is the same, but the lengths (±365 and ±381 mm) are longer than those of the Coulex chamber (±351.5 and ±367.5 mm). Point (See fig. 5) 0 1 2 3 4 5 6 7 8
2 The
xM B , yM B , zM B [mm] 0.00, 0.00, 0.00 -127.50, -381.00, 80.00 -127.50, 381.00, 80.00 127.50, -381.00, 80.00 127.50, 381.00, 80.00 -127.50, -365.00, 80.00 -127.50, 365.00, 80.00 127.50, -365.00, 80.00 127.50, 365.00, 80.00
xCCS , yCCS , zCCS , HCCS [m] 1864.28940, 2282.82486, 2437.48881, 437.49215 1864.68869, 2282.78037, 2437.56881, 437.57214 1863.94375, 2282.62005, 2437.56881, 437.57216 1864.63504, 2283.02966, 2437.56881, 437.57215 1863.89010, 2282.86935, 2437.56881, 437.57216 1864.67305, 2282.77700, 2437.56881, 437.57214 1863.95939, 2282.62342, 2437.56881, 437.57216 1864.61940, 2283.02630, 2437.56881, 437.57215 1863.90574, 2282.87271, 2437.56881, 437.57216
nominal target position is taken from version 1.1 of the EDMS document 1323585.
8
Acces PDF Wild T1a Theodolite Manual exercise just what we find the money for under as without difficulty as evaluation wild t1a theodolite manual what you as soon as to read! DailyCheapReads.com has daily posts on the latest Kindle book deals available for download at. Read Online Wild T1 Theodolite Manual Wild T1 Theodolite Manual Recognizing the showing off ways to acquire this books wild t1 theodolite manual is additionally useful. You have remained in right site to begin getting this info. Acquire the wild t1 theodolite manual associate that we manage to pay for here and check out the link. Here 's an example out of the Geodesy folder. It is the first page of the 'subtense bar'. According to the list it was first produced as T7 1929.Starting in 1956, then as BL (BasisLatte) 1966 as BL21 and from 1967 to 1985 as GBL3 (Geo-BasisLatte version 3). Wild T1a Theodolite Manual Get Free Wild T1 Theodolite Manual The Wild T1 is a Double Centre Theodolite with two independend cylindrical axes and they where produced between 1933 and 1996 in mainly four versions as shown abouve. Wild T1 - Virtual Archive of Wild Heerbrugg This page will give you a. Maintenance Manual Theodolite, Surveying, Directional, One Minute (Wild-Heerbrugg Model T16-75DEG) NSN 6675-014-075-3278 Theodolite, Surveying, Directional, 0.2 Mil (Wild-Heerbrugg Model T16-84MIL) NSN 6675-01-191-4777 Approved for public release; distribution is unlimited TM 5-6675-312-14, dated 20 June 1980, is changed as follows.

Theodolite vs Transit
How to Use a Theodolite
How a Theodolite Works
Types of Theodolites
There are two different kinds of theodolites: digital and non digital. Non digital theodolites are rarely used anymore. Digital theodolites consist of a telescope that is mounted on a base, as well as an electronic readout screen that is used to display horizontal and vertical angles. Digital theodolites are convenient because the digital readouts take the place of traditional graduated circles and this creates more accurate readings.
Parts of a Theodolite
Like other leveling instruments, a theodolite consists of a telescope mounted on a base. The telescope has a sight on the top of it that is used to align the target. The instrument has a focusing knob that is used to make the object clear. The telescope contains an eyepiece that the user looks through to find the target being sighted. An objective lens is also located on the telescope, but is on the opposite end as the eyepiece. The objective lens is used to sight the object, and with the help of the mirrors inside the telescope, allows the object to be magnified. The theodolite's base is threaded for easy mounting on a tripod.

How Does a Theodolite Work?
A theodolite works by combining optical plummets (or plumb bobs), a spirit (bubble level), and graduated circles to find vertical and horizontal angles in surveying. An optical plummet ensures the theodolite is placed as close to exactly vertical above the survey point. The internal spirit level makes sure the device is level to to the horizon. The graduated circles, one vertical and one horizontal, allow the user to actually survey for angles.
How to Use a Theodolite
- Mark the point at which the theodolite will be set up with a surveyor’s nail or a stake. This point is the basis for measuring angles and distances.
- Set up the tripod. Make sure the height of the tripod allows the instrument (the theodolite) to be eye-level. The centered hole of the mounting plate should be over the nail or stake.
- Drive the tripod legs into the ground using the brackets on the sides of each leg.
- Mount the theodolite by placing it atop the tripod, and screw it in place with the mounting knob.
- Measure the height between the ground and the instrument. This will be used a reference to other stations.
- Level the theodolite by adjusting the tripod legs and using the bulls-eye level. You can make slight tunings with the leveling knobs to get it just right.
- Adjust the small sight (the vertical plummet) found on the bottom of the theodolite. The vertical plummet allows you to do ensure the instrument remains over the nail or stake. Adjust the plummet using the knobs on the bottom.
- Aim the crosshairs in the main scope at the point to be measured. Use the locking knobs on the side of the theodolite to keep it aimed on the point. Record the horizontal and vertical angles using the viewing scope found on the theodolite’s side.
Theodolite vs Transit Level
A theodolite is a precision instrument used for measuring angles both horizontally and vertically. Theodolites can rotate along their horizontal axis as well as their vertical axis. Theodolites have a lot in common with transits.
A transit is a surveying instrument that also takes accurate angular measurements. Along with the transit, theodolites have mounted telescopes that can be rotated in different directions. Both theodolites and transits can be used for similar projects, but there are slight differences between the two instruments. Transits use vernier scales and external graduated metal circles for angular readings. Theodolites use enclosed graduated circles and angular readings are taken using an internal magnifying optical system. Theodolites tend to have a more precise reading and provide greater accuracy in measuring angles than transits do.
Theodolites are mainly used for surveying, but they are also useful in these applications:
- Navigating
- Meteorology
- Laying out building corners and lines
- Measuring and laying out angles and straight lines
- Aligning wood frame walls
- Forming panels
- Plumbing a column or building corner
Advantages of Using a Theodolite
Theodolites have many advantages when compared to other leveling instruments:
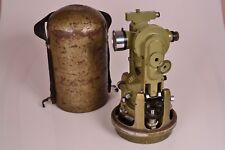
- Greater accuracy.
- Internal magnifying optical system.
- Electronic readings.
- Horizontal circles can be instantly zeroed or set to any other value.
- Horizontal circle readings can be taken either to the left or right of zero.
- Repeat readings are unnecessary.
Theodolites have an internal optical device that makes reading circles much more accurate than other instruments. Also, because the theodolite allows you to take fewer repeat readings, these measurements can be made much more quickly. Theodolites with optical instruments have advantages over other layout tools. They have more precise measurements, they are unaffected by wind or other weather factors, and they can be used on both flat ground and sloped ground.
Wild T1a Theodolite Manual Software
Caring For a Digital Theodolite and Helpful Hints
Like other instruments, theodolites require proper care and maintenance to ensure the best results and reduce wear and tear on the instrument.
- Do not submerge instrument in water or any other chemical.
- Do not drop instrument.
- Make sure theodolite is locked in its case while transporting.
- When raining, use cover over instrument.
- Do not look directly into the sunlight through the telescope on the instrument.
- Using a wooden tripod can protect the instrument from vibrations better than an aluminum tripod would.
- Using the sunshade attachment is important; any sudden temperature changes can cause incorrect readings.
- Never hold the instrument by the telescope.
- Always have a substantial level of battery power on your instrument.
- Always clean the instrument after using.
- Dust in the case or on the instrument can cause damage.
- If the theodolite is damp or wet, allow it time to dry out before storing it in its case.
- When storing, make sure that the telescope on the instrument is in the vertical position.
- When the theodolite is being re-leveled, the position over the ground point must be checked and rechecked to ensure the same position.
- When the theodolite is being repositioned over a ground point, the level must be checked and rechecked to ensure its accuracy.
If you’d like more how-to information, visit Johnson Level’s complete list of how-to guides for tools and levels.
Wild T1 Theodolite Manual Pdf
Shop theodolites, builder's levels and other optical instruments.
©2015 Johnson Level & Tool Mfg. Co., Inc.
Electronic Digital Theodolite - Two Second 40-6932 | Electronic Digital Theodolite - Five Second 40-6935 | Five-Second Electronic Digital Theodolite with Laser |